Heatmap of Energy
Consumption
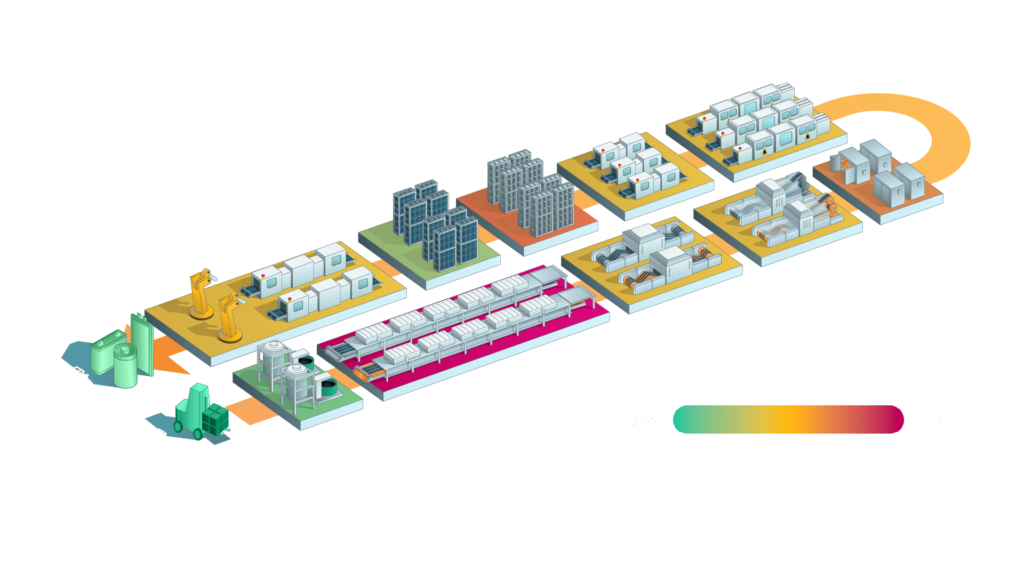
Incoming Quality Control
Energy Consumption
The costs associated with the process step of Incoming Quality Control in battery cell production are crucial for overall efficiency and production quality. Through meticulous inspection and testing of incoming materials and components, manufacturers can detect potential defects or quality issues early, thereby avoiding costly rework or scrap. An effective IQC strategy enables companies to optimize their production costs by reducing failures, improving product quality, and maximizing the overall performance of their battery cells. The process itself has a limited impact on the overall cost structures in battery cell production.
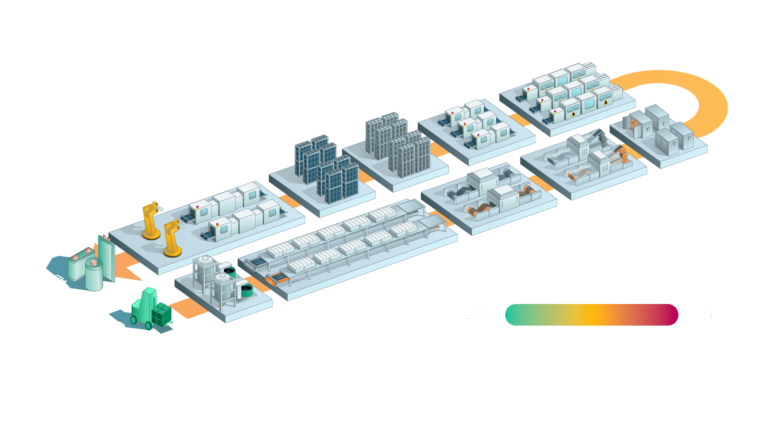
Dosing & Mixing
Energy Consumption depending on mixing method
The dosing and mixing process in lithium-ion-battery manufacturing can significantly impact energy consumption. The slurry mixing step contributes to the total manufacturing cost and takes a relatively long time to prepare a suitable slurry for subsequent manufacturing processes. The energy consumption during mixing is influenced by the mixing method and sequence.
Innovative mixing technologies such as 3D hydrodynamic shear mixing, ball milling, and ultrasonic mixing have been explored to improve mixing uniformity and efficiency. For instance, ultrasonic mixing has been found to increase mixing uniformity for dry powder mixing and high-concentration slurry, potentially reducing energy consumption. However, the cost and reliability of large-scale implementation of these technologies need to be evaluated before industrialization. [1]
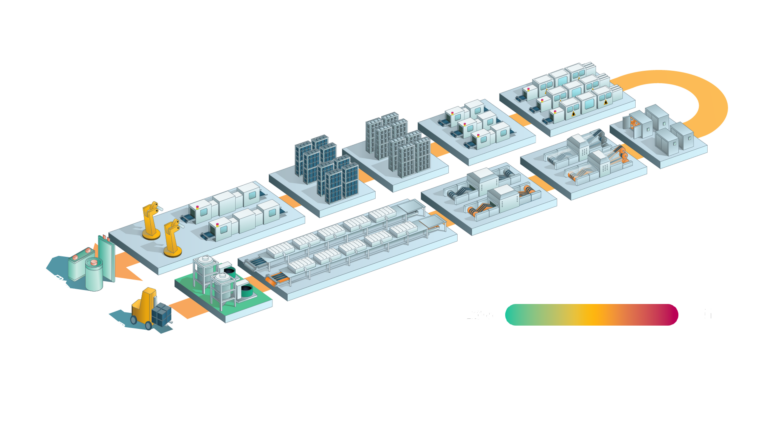
Coating & Drying
The energy guzzler
Coating and drying are identified as two of the main energy consumers in the production of lithium-ion and post lithium-ion-battery cells. In the present scientific context, the energy consumption of coating and drying processes is emphasized as one of the most substantial sources of natural gas consumption in the production process of battery cells. It is emphasized that the high energy demand is attributable to the elevated temperature of up to 150°C for a longer period within the oven. In addition, exhaust gas cooling is an essential part of the high energy consumption. The operation of the dry rooms in particular require a considerable amount of natural gas, primarily for the regeneration of the dehumidification unit. In combination, these processes significantly contribute to the total energy and natural gas consumption in the production of battery cells.
Degen (2022) discusses the potential impact of new technologies on energy consumption, including the use of heat pumps, learning effects, and economies of scale, which are projected to decrease the energy consumption for future production of LIB and PLIB cells. It is shown that when new technologies are fully industrialized and applied by 2040, significant savings in energy consumption per produced and processed electrode area can be achieved. [4,5]
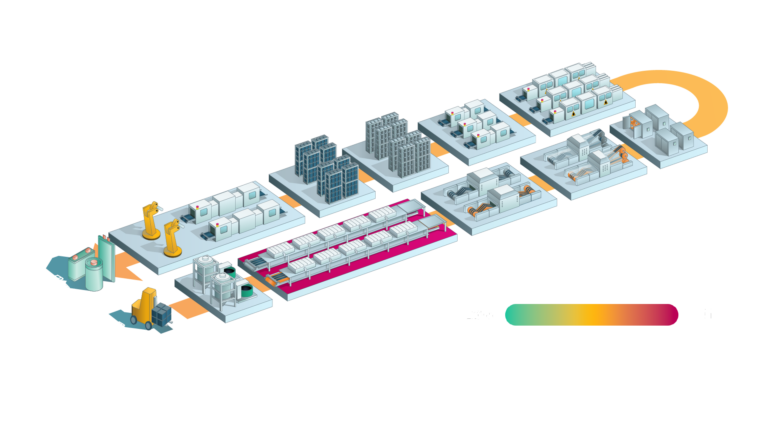
Calendering
Requires exact parameters for optimum utilization of energy
Degen (2022) identifies calendering as an energy-intensive process in the production of lithium-ion and post lithium-ion battery cells. Calendering is a process that involves compressing the electrode material to improve its mechanical properties and increase its energy density. The calendering process can be energy-intensive, which is influenced by the calendering temperature and other parameters. Research has shown that applying higher rolling temperature during calendering can improve the elastic deformability of the thermoplastic binder, such as PVDF, and lead to lower porosity and compaction resistance and higher adhesion strength. However, calendering defects can occur if incorrect parameters are applied, leading to energy waste and material waste.
To optimize the calendering process and reduce energy consumption, researchers have explored numerical simulation and artificial intelligence (AI) to modify the manufacturing parameters. For example, the discrete element method (DEM) was used to analyze the behavior of electrodes during the calendering process and predict the elastic recovery after pressure release. Additionally, a 3D physics-based model has been developed to calculate electrode properties, which can be used to train and validate machine learning algorithms to identify the interdependencies between calendering parameters and electrode properties. [1,4]
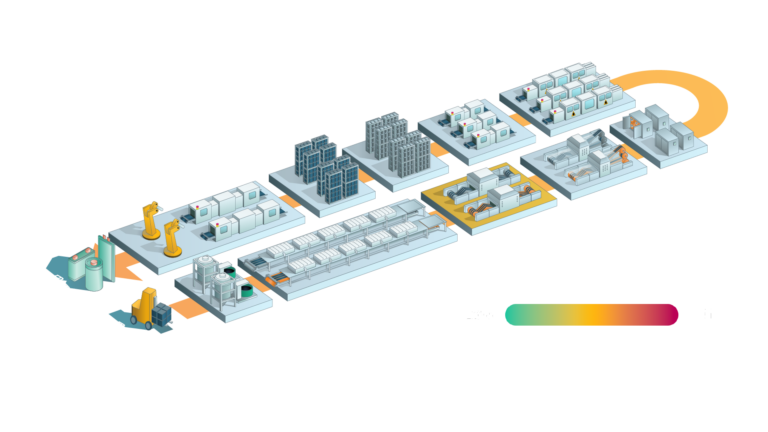
Slitting
Reduction of edge defects to reduce energy consumption
The slitting process has a relatively low impact on energy consumption compared to other manufacturing processes. Slitting is a step in the roll-to-roll operation to prescribe electrode width after calendering and it is a low-cost and high throughput process with established techniques.
Nevertheless, the edge defects such as burr and dross may penetrate the separator and cause a short circuit, which can lead to material waste and energy waste. Therefore, optimizing the slitting process to reduce edge defects can contribute to reducing energy consumption indirectly by reducing material waste. [1]
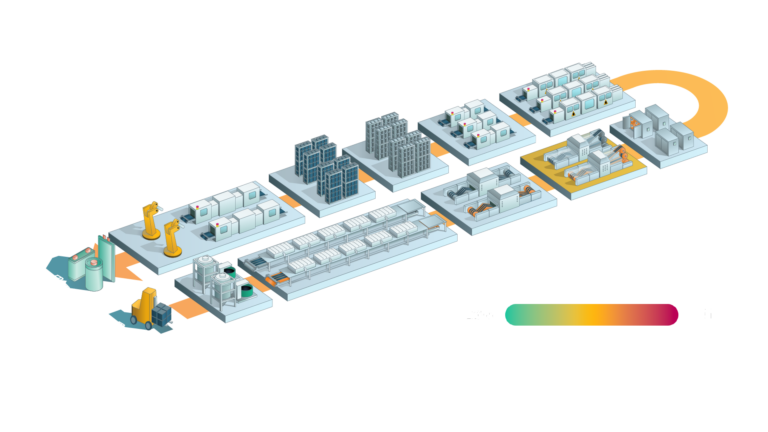
Vacuum Drying
A major energy consumer
The vacuum drying process in lithium-ion-battery manufacturing has a significant impact on energy consumption. Vacuum drying is a necessary process that removes residual moisture from the electrode material to prevent the formation of hydrogen fluoride gas, which can damage the anode material and cause safety concerns.
The energy consumption is influenced by the drying temperature, pressure, and time. Research has shown that the drying temperature and pressure can affect the drying rate and energy consumption . To reduce energy consumption during vacuum drying, researchers have explored alternative drying methods such as infrared ray and laser beam heating. For example, applying a 450-W/1,070-nm fiber laser can halve the drying energy consumption. [1]
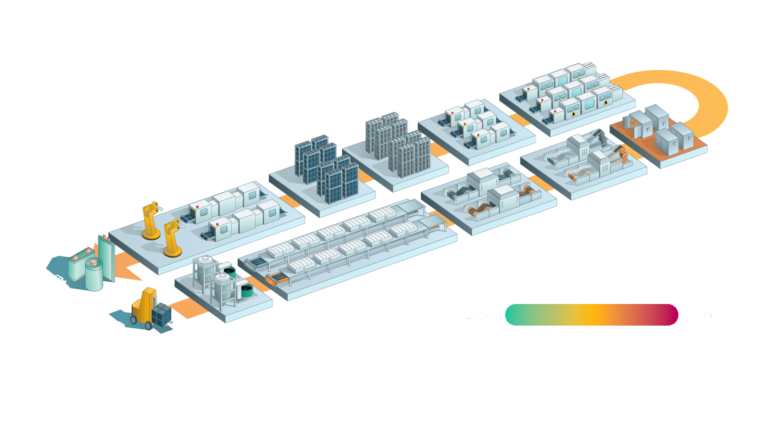
Assembly
Diffrent systems with different energy consumption
The energy consumption during the assembly process is influenced by factors such as the need for maintaining low moisture levels, the use of solvents, and the overall efficiency of the assembly operations. In addition, the assembly process requires strict control of moisture levels to ensure the quality and performance of the battery cells. Maintaining low moisture levels in the assembly environment leads to energy consumption, especially in facilities where specialized climate control systems are employed to meet these requirements.
Some assembly processes may involve the use of solvents for tasks such as electrode coating or other manufacturing operations. The use of solvents can contribute to energy consumption, particularly during drying and solvent recovery stages.
The overall efficiency of the assembly operations, including the handling of materials, equipment utilization and process optimization, can impact the energy consumption. Improving the efficiency of assembly processes can help reduce energy consumption and enhance the sustainability of LIB manufacturing. More efforts to reduce energy consumption during the assembly process may involve the development of innovative technologies such assolvent-free coating, improved moisture control systems, and optimized manufacturing workflows. These initiatives can lead to lowering the environmental impact and operational cost. [1]
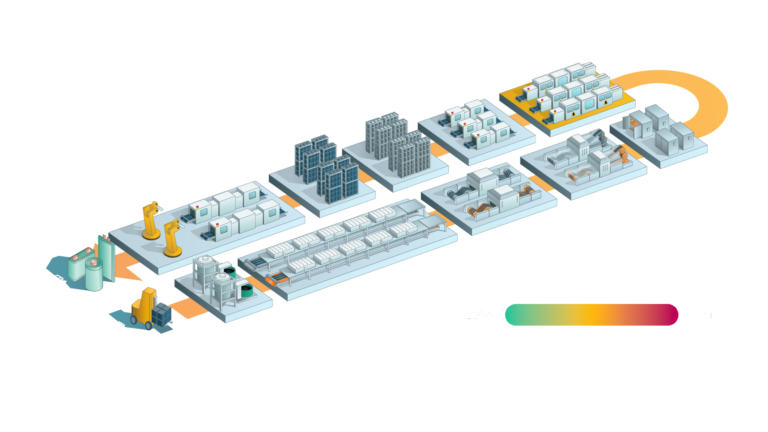
Electrolyte Filling
Energy consumption as a result of machinery, electrolyte and efficency
The energy consumption during the electrolyte filling process is influenced by factors such as the electrolyte, the filling method, and the overall efficiency of the filling operations. The type of electrolyte used in LIB manufacturing impact the energy consumption during the filling process. For example, some electrolytes may require heating or cooling to achieve the desired viscosity.
The overall efficiency of the filling operations, including the handling of materials, equipment utilization and process optimization, can impact the energy consumption. Improving the efficiency of filling processes helps reducing energy consumption and enhance the sustainability of LIB manufacturing. In addition, efforts to reduce energy consumption during the electrolyte filling process may involve the development of innovative technologies for electrolyte preparation, filling methods and optimized manufacturing workflows. [1]
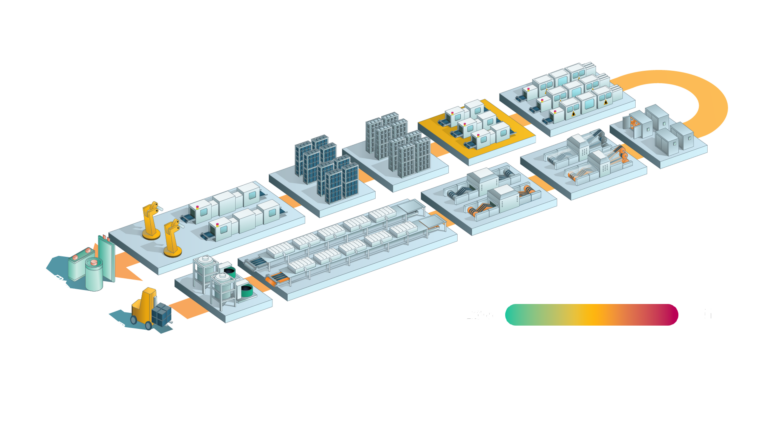
Formation
Charge and discharge with electrical energy
The formation process step is one of the main energy drivers along with coating and drying. The formation process involves cycling the battery cells through charge and discharge cycles to activate and stabilize their electrochemical properties. The efficiency of the charging and discharging equipment has an impact on the overall energy consumption.
Maintaining specific temperature conditions during the formation process is important for achieving desired battery performance. Heating or cooling systems used to control of the cells during contribute to energy consumption.
Efforts to reduce energy consumption during the formation process may involve the development of advanced formation protocols, energy-efficient temperature control systems and optimized charging and discharging strategies. Additionally, innovations in battery chemistry and design may lead to more efficient formation processes, reducing energy consumption and improving the overall sustainability of LIB manufacturing. [1,5]
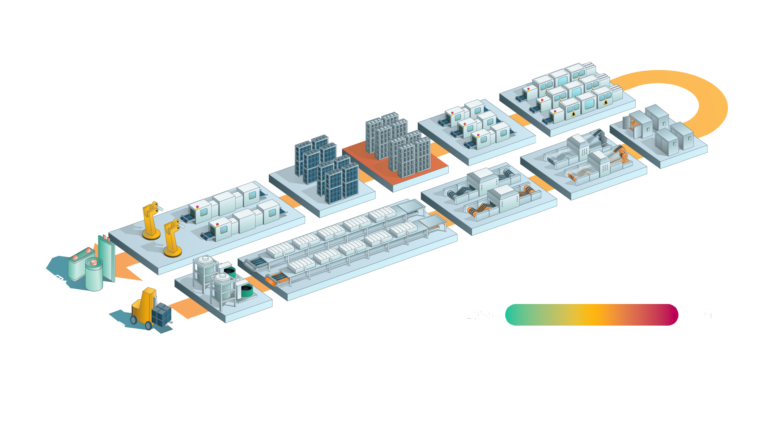
Aging
Energy consumption as in real use
The aging process involves subjecting the battery cells to controlled conditions to simulate real-world usage and assess their longterm performance. Similar to the formation process, maintaining specific temperature conditions during the aging process is essential. Heating or cooling systems used to control the temperature influence the overall energy consumption. Extended aging periods may result in higher energy usage, especially if the process involves maintaining specific temperature and cycling conditions over an extended period. Energy consumption may also be influenced by the use of monitoring and testing equipment during the aging process. Instruments used to measure the performance and characteristics of the cells may contribute to overall energy usage.
Efforts to reduce energy consumption during the aging process involve the development of advanced aging protocols, energy-efficient temperature control systems, and optimized monitoring and testing methodologies. Furthermore, innovations in battery chemistry and design lead to more efficient aging processes. [1]
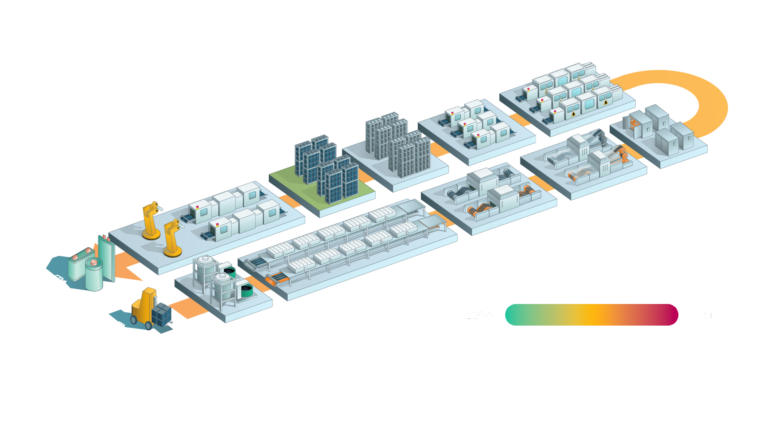
End-of-line Quality Control
Energy consumption and quality
The End-of-line quality control process involves the inspection and testing of finished battery products to ensure they meet specified quality and performance standards. The use of testing equipment, including instrumentation for electrical, mechanical, and performance testing, lead to energy consumption during the quality control process.
Energy may be consumed by the data analysis and processing systems used to interpret and evaluate the results of quality control tests. Maintaining specific environmental conditions, such as temperature and humidity, within the quality control area to ensure accurate testing and measurement can contribute to energy consumption.
Efforts to reduce energy consumption during the End-of-line quality control process may involve the development of energy-efficient testing equipment, optimized data analysis and processing systems and sustainable environmental control measures. Additionally, the integration of advanced automation and robotics technologies can lead to more efficient and energy-conscious quality control processes, reducing energy consumption, and improving the sustainability of LIB manufacturing. [1]
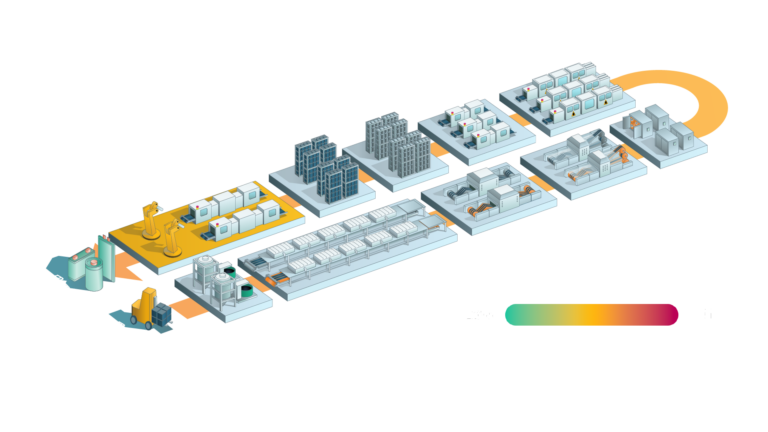
Outgoing Quality Control
Minor impact on use of energy
The energy consumption in the process step of outgoing quality control has a minor impact on the overall consumption of battery cell production, yet it is influenced by several factors. These factors include the type and efficiency of the testing equipment used, as well as the complexity and duration of the tests conducted. Furthermore, the utilization of automated testing procedures plays a role in reducing energy consumption. External factors such as ambient temperature and humidity can also influence the energy demand for conditioning and operating the testing equipment. A holistic consideration of these factors is important for optimizing energy consumption in OQC and improving the overall efficiency of battery cell production.
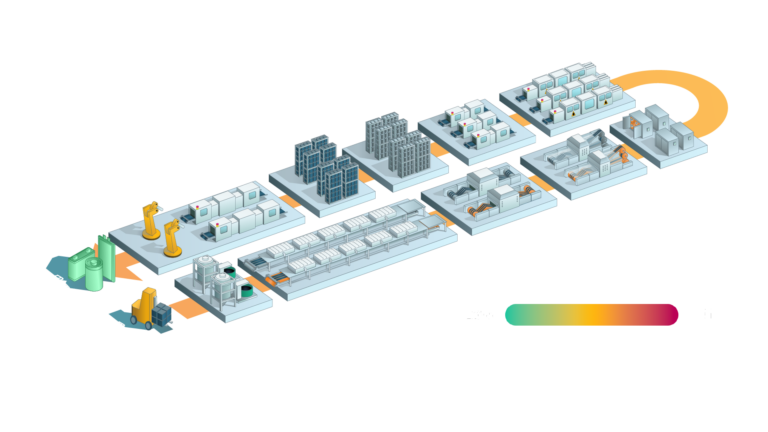
Source:
[1] Liu,Y.; Zhang, R.; Wang, J.; Wang,Y (2021): Current and future lithium-ion battery manufacturing
[2] Orangi, Sina; Hammer Stromann, Anders (2022): A Techno-Economic Model for Benchmarking the production cost of Lithium-Ion Battery cells
[3] Mauler, Lukas; Duffner, Fabian; Leker, Jens (2021): Economies of scale in battery cell manufacturing: The impact of material and process innovations.
[4] Degen, F.; Schütte,M. (2021): Life cycle assessment of the energy consumption and GHG emissions of state-of-the-art automotive battery cell production
[5] Degen, F.; Winter, M.; Bendig, D.; Tübke, J. (2022): Energy consumption of current and future production of lithium-ion and post lithium-ion battery cells